Electrolyte Addition for Enhanced Wastewater Treatment by Electrolysis using Cu Electrode
Article information
Abstract
In this study, the effect of electrolyte addition on the removal of organics and nutrients in electrochemical wastewater using a copper electrode, and the characteristics of the by-product of electrolysis were investigated. The removal of organics increased significantly as shorter reaction times upon the addition of chloride ion, and most of the electrolysis reaction was completed within 20 min. The reaction rate gradually increased in proportion to the Cl−/COD ratio, whereas the highest removed mass of organic matter per mass of added electrolyte was observed at a Cl−/COD ratio of 1. After the addition of electrolyte, significant removal of ammoniacal nitrogen was observed as a result of the enhanced generation of oxidizers such as hypochlorite. Excellent phosphorus removal was also achieved in a very short reaction time (within 2 min) by electro-coagulation. As the electrolysis progressed, the amount of by-product increased gradually, whereas a decrease of sludge volume index was observed after the addition of electrolyte. This indicated that the settling performance of the by-products was better, and their removal would be easily achieved.
1. Introduction
Wastewater is defined as used water discharged from residences, institutions, and commercial or industrial facilities. Untreated wastewater contains numerous pathogenic microorganisms, and the decomposition of the organic matter it contains will lead to nuisance conditions. Wastewater also contains nutrients that can stimulate the growth of aquatic plants, and may contain toxic or potentially carcinogenic compounds. For these reasons, the immediate and nuisance-free removal of wastewater is necessary to protect public health and the environment [1]. Biological nutrient removal (BNR) has been commonly used for the removal of nutrients from wastewater. However, this method has disadvantages such as operating complications by internal sludge and nitrate recycling, continuous need of air supply, high operating costs, sensitivity to shock toxic loadings, longer reaction time for the treatment, and high sensitivity of microorganisms to pH and temperature [2].
Using electricity to treat water and wastewater is very convenient to extirpate pollutants involving toxic organic compounds and extremely small particles [3]. With the strict environmental regulations regarding wastewater discharge in recent years, electrochemical technology has been widely utilized in the treatment of various types of wastewaters such as textile [4-6], oil and oil-in-water emulsion [7-9], laundry [10-12], livestock [13-15], leachate [16-18], and sewage [2,19-20]. The use of electrochemical technology provides several benefits, such as strong oxidation, easy operation, no limitation by a seasonal variation, variable capability to influent flux and quality, and rapid treatment time, over the conventional wastewater treatment methods.
In general, electrochemical removal of pollutants in wastewater can be achieved by electro-coagulation or electro-oxidation. Pollutants such as suspended particles and matter containing organics and nitrogen in wastewater can be removed by electrocoagulation, separating the coagulated and flocculated contaminants with eluted ions from electrodes [21]. Electro-oxidation can be defined as an electrochemical reaction involving direct oxidation on the anodes or indirect oxidation using by-products such as hypochlorite (HOCl), and is an invaluable method for the removal of soluble contaminants [16, 22].
Most electrolysis methods use a pair of metallic electrodes, and because the electrochemical treatment depends on the pollutants in wastewater, the choice of electrode material is crucial. In electrooxidation, boron doped diamond electrodes (BDD) and titanium electrodes electroplated with metal oxides such as SnO2, PbO2, and TiO2 were generally utilized [22-24]. Iron and aluminum electrodes are also used in electro-oxidation, but especially for electro-coagulation. In general, aluminum and iron are used as a relatively active anode in combination with a relatively stable cathode such as titanium [21].
In a previous study [25], various electrodes such as aluminum, iron, and copper were applied to treat refractory wastewaters, of which the copper electrodes displayed high efficiency. The redox potentials of aluminum and iron are −1.662V (for Al ↔ Al3+ + 3e−) and −0.440V (for Fe ↔ Fe2+ + 2e−), respectively, while for copper it is +0.337V (for Cu ↔ Cu2+ + 2e−). A more negative potential implies that the electrode is more active, and thus it can be easily oxidized and corroded [26]. On the contrary, an electrode with a more positive potential such as copper, can be maintained and managed without the formation of surface scale and quick exhaustion [25]. Thus, the removal of suspended matter in water and wastewater by copper could be achieved successfully, but like aluminum or iron electrodes, it had limitations in the treatment of dissolved pollutants [27, 28].
The electro-oxidation of dissolved pollutants in water and wastewater can be promoted by amplification of the energy, such as the electric current or voltage, which flows through the impurities in water. However, precautions must be taken to avoid electric shock injury accidents. Several studies have been performed to increase the electric current and improve the electro-oxidation efficiency by addition of an electrolyte supplement [29-31]. Salt is usually employed to increase the conductivity of the water or wastewater to be treated. The supplement by salt can lead to improved electrical conductivity in the water, as well as an increase of electric current by the reduction of resistance. This technique can effectively oxidize many inorganic and organic pollutants at high chloride concentration. If the chloride content in the raw wastewater is low, a large amount of salt must be added to increase the process efficiency [32-35]. In this study, the effect of electrolyte supplement on the removal of organics and nutrients in electrochemical wastewater treatment using a copper electrode was evaluated. We have also investigated the characteristics of the by-products of increasing the electric current.
2. Experimental Section
The apparatus used in this study was composed of a reactor of ~250 mL capacity and a pair of copper electrodes with a power supply, as presented in Fig. 1. The length of each electrode with a width of 10 mm was 190 mm, and most of the electrode was submerged in synthetic wastewater. The electrode spacing (distance between anode and cathode) was 7.5 mm, and the pair of electrodes was fixed by a plastic clamp.
Two types of synthetic wastewater were prepared and used: type A contained organic matter only, and type B contained nutrients as well as organics. The wastewater of type A with organic matter concentration of 200 mg/L as chemical oxygen demand (COD) and various electrolyte concentrations of 200 mg/L, 400 mg/L, and 800 mg/L, was prepared by solution of C6H12O6 and sodium chloride (NaCl) in distilled water, and used for the derivation of optimal chloride to organics (Cl−/COD) ratio for the enhanced removal of organics. Once the optimal chloride to organics ratio identified using type A, further experiments were conducted using type B wastewater. The concentrations of organics, nitrogen, and phosphorus in wastewater of type B were 200 mg/L, 40 mg/L, and 10mg/L, representing the average concentration of general domestic wastewater [36-41].
Experiments were conducted in batches. Electrochemical experiments were also performed under a constant voltage of 24V and a variable current with an upper limit of 10A. During the electrolysis, the current value between the anode and cathode was measured simultaneously by a digital multi measuring instrument (SEAHAN, SH-852). All analyses were conducted as per procedures in the American Public Health Association (APHA) Standard Methods [42]. The supernatants of the effluents after settling for 30 min were collected as a sample for the evaluation of the electrochemical treatment. The by-product of electrolysis was dried and analyzed by scanning electron microscopy (SEM; COXEM, EM-30) and energy dispersive spectroscopy (EDS; BRUKER, XFlash Detector 410-M).
3. Results and Discussion
Pollutants in wastewater can be generally removed by electro-oxidation or electro-coagulation [21,22]. In our previous study [25], most organic matter could be removed in the electrolysis process by electro-coagulation detaching by-products formed on the surface of the anode, and not separating coagulated and flocculated contaminants with eluted ion from electrodes. In this study, therefore, we investigated the effect of electrolyte addition only on the removal of COD representing the organic pollutants in wastewater with chloride ions as the electrolyte.
Electro-oxidation of organic pollutants in wastewater can occur by indirect oxidation or direct anodic oxidation. During electrochemical oxidation, the removal of organic pollutants was considered to be achieved by indirect oxidation, utilizing the hypochlorite ion and hypochlorous acid formed by the anodic oxidation of chlorine in the synthetic waste-water. A series of reactions involving indirect oxidation are shown in equations (1)-(5).





The COD removal could be improved by the addition of chloride ions during the electrochemical process, as illustrated in Fig. 2. Costa and Olivi [43] have also reported that the addition of electrolyte to the solution resulted in the improved organic oxidation rate due to the participation of electro-generated hypochlorite ions. At a chlorine ions to organics ratio (Cl−/COD) of 2, the highest COD removal could be achieved regardless of the reaction time. Higher COD removal was also obtained after 5 min at a Cl−/COD ratio of 1, whereas relatively lower COD removal was observed for 10 min when the Cl−/COD ratio was 4. Table 1 shows the reaction rate constants and energy efficiency for different Cl−/COD ratios. For the calculation of rate constants, it was assumed that reactive organic matter decomposes at an overall rate directly proportional to its own concentration during electrochemical treatment (i.e., by first-order kinetics). The values of the reaction rate constants also increased gradually, as the chloride ions to COD ratio increased, and the highest value was observed at a Cl−/COD ratio of 4. In the absence of added chloride ions, the reaction did not follow the firstorder kinetics, and a relatively poor oxidation of organic matter was observed (Fig. 2). Thus, in order to improve the removal of organic matter by electrochemical treatment, the appropriate amount of added chloride ions is necessary, and it can be calculated from the removed mass of organic matter per mass of electrolyte addition. As presented in Table 1, the optimal COD removal per added electrolyte was obtained at a Cl−/COD ratio of 1. On the other hand, the energy efficiency decreased dramatically, as the chloride ion to COD ratio increased, showing that energy loss occurs upon electrolyte addition. From these results, a chloride to organics ratio of 1 was used to evaluate the effect of electrolyte addition for the removal of organics and nutrients by electrochemical wastewater treatment using a copper electrode.
Synthetic wastewater including organic materials as well as nutrients, representing the typical concentration of wastewater from separate sewers in Korea, was used to evaluate the effect of chloride ion addition on the removal of organic matter (Fig. 3). For comparison, experiments were also carried out in the absence of added chloride ions. As the electrolysis time increased, the overall organic matter removal increased, through the electrolysis procedure. In the absence of electrolyte addition, the organic matter was not removed during the initial electrolysis time for 5 min after which the removal gradually increased. The organic matter removal increased noticeably upon the addition of chloride ions even at short reaction times, and most electrolysis reactions were completed within 20 min. After 20 min, the values of current density, pH, and Oxidation Reduction Potential (ORP) were almost unchanged despite the electrolysis procedure.
In electrolysis carried out at a constant voltage, the current density can reduce due to the decrease of the electrical conductivity in wastewater. The electrical conductivity is the reciprocal of electrical resistivity, and measures a material's ability to conduct an electric current [42]. The electrical conductivity is also proportional to the concentration of electrolyte ions. Hence, the changes in the electrolyte ions in water and wastewater can be observed by monitoring the current density. The plot of the current changes with the reaction time of electrolysis (Fig. 4) showed that the values of current density decreased gradually. When the chloride ions were added, the current density was approximately doubled. The decrease in the current densities at Cl−/COD ratios of 0 and 1, at a reaction time of 60 min were 27.4 and 37.0 A/m2, respectively. This implies that the reduction in the concentration of chloride ions affecting the removal of organic matter, and the conversion reaction from chloride to chlorine in wastewater as presented in equation (1) occurs actively, upon the addition of electrolyte. The current densities reduced only slightly after 20 min, because most of the electrolysis reaction was complete.
During the electrolysis of wastewater, hydrogen gas (H2) and hydroxide (OH−) are produced at the cathode, because there are many electrolytic ions with a lower standard potential than hydroxide and hydrogen. For this reason, the concentration of hydroxide ion at the cathode would increase, and possibly the pH value as well. The increase of pH value by the hydroxyl ion formed at the cathode can be explained in terms of change in the ORP by electrolysis. From the ORP, it is possible to infer if the main reaction in water is oxidation or reduction. The plot of pH value and ORP vs. the addition of electrolyte (Fig. 5) during electrolysis, showed an increase in the pH value and a decrease in the ORP. A higher pH value was also observed after the addition of chloride ions. The variation of pH and ORP could be attributed to the generation of hydroxyl ions and hydrogen gas, since electrolysis results in the decomposition of water at the cathode. The ORP value further declined due to the high reactivity as a result of electrolyte addition.
Copper ions can be eluted consistently from the anode during electrolysis using a copper electrode, and the by-products such as Cu(OH)2, Cu2O, and CuO are generated as shown.




In electrochemical treatment, the final effluent should be discharged after separation of the solids from the liquid; hence, the settling characteristics of the by-products (sludge) which contain pollutants or eluted electrode is very important. In this study, the settled volume of sludge after 30 minutes (SV30) and the sludge volume index (SVI) were used to evaluate the settling characteristics of the by-products (Fig. 6). The SV30 was determined by placing the sample in a 1-2 L cylinder and measuring the settled volume after 30 min. SVI is the suspended solid concentration of the sample corresponding to SV30. As the electrolysis progressed, the amount of by-products increased gradually, most notably after the addition of electrolyte. However, decrease of SVI was observed after the addition of chloride ions. A lower value of SVI generally indicates better settling characteristics of the by-products. This indicates that removal of the by-products of electrolysis would be easily achieved.
The color of the by-products changed from blue to dark brown during electrolysis. In general, the colors of the copper by-products are black (CuO), red (Cu2O), and green blue (Cu(OH)2 or Cu3(PO4)2) [44]. The by-product was dried and analyzed by energy dispersive spectroscopy (EDS), and the sludge produced through initial electrolysis contained 18.66 at. % of copper, 55.12 at. % of oxygen, and 25.48 at. % of carbon. This implied a combining ratio for Cu:O above 1:2, and hence, the by-products are likely to Cu(OH)2 and Cu3(PO4)2. However, the final by-product of electrolysis consisted of 46.68 at. % of copper, 52.41 at.% of oxygen, along with a small amount of carbon. From this result, the form of the final by-product was believed to be CuO. This indicates that the copper ions were oxidized and reacted with the hydroxide ions in the beginning of the electrolysis, and reacted with oxygen after the completion of electrolysis. The scanning electron microscope (SEM) images of the microstructure of the by-product by SEM are shown (Fig. 7), displaying the loose binding of the particles.
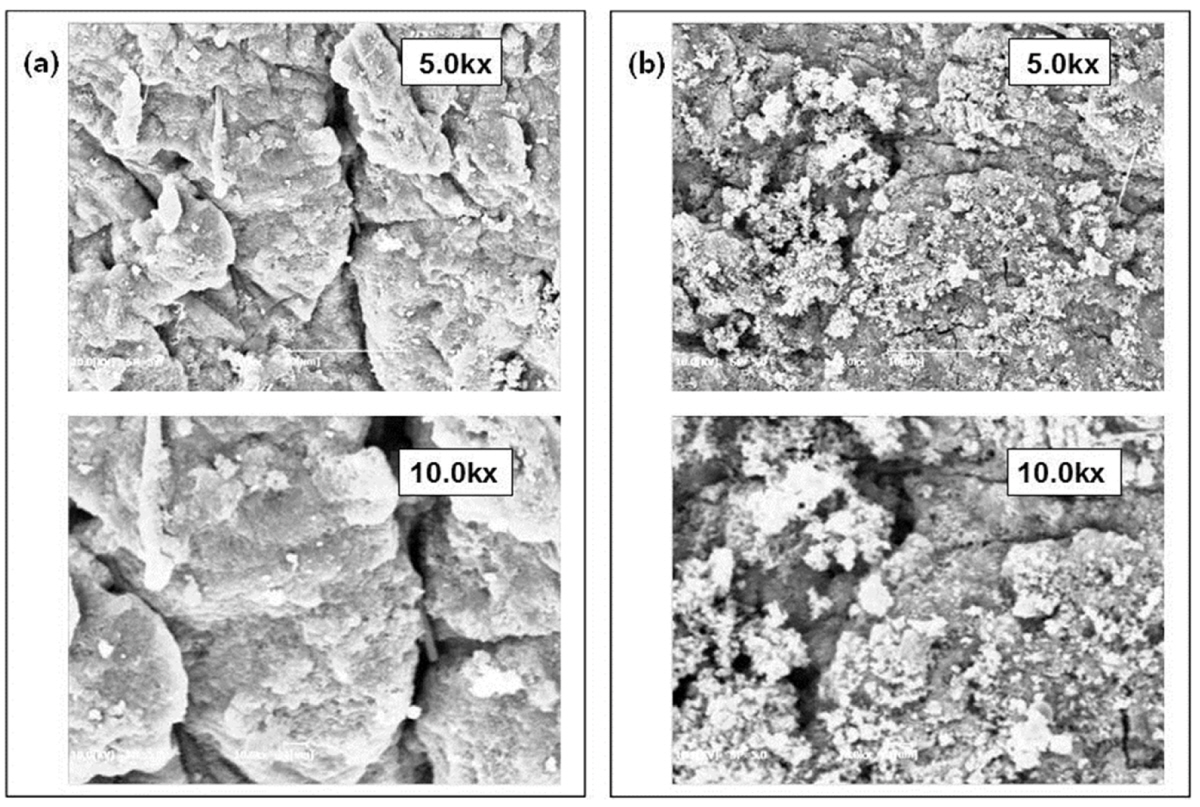
SEM images of the by-product of electrolysis with copper electrode (a) within 30 min and (b) after 60 min.
Nitrogen in wastewater can also be removed by electro-oxidation using by-products such as hypochlorite. Chloride in aqueous environment is converted into chlorine by electrolysis, and the ammoniacal nitrogen can be oxidized by the hypochlorite generated from dissolved chlorine [45], as shown.

In this study, chloride ions were added for the enhancement of nitrogen oxidation as well as the removal of organic matter, and Fig. 8 shows the variation of removed ammoniacal nitrogen during electrolysis. The removal of ammoniacal nitrogen significantly increased after the addition of chloride ions. In the absence of electrolyte addition, the ammoniacal nitrogen was not removed in the initial 5 min of electrolysis. However, higher nitrogen removal was achieved after the addition of chloride ion. Oxidizer such as hypochlorite could be generated in a shorter reaction time by the additional supplement of chloride ion, and this result was similar to that of Li et al. [45] and Cho et al. [30]. In electrolysis, phosphorus can be extirpated by electrocoagulation through the formation of compounds such as copper phosphate (Cu3(PO4)2). From the plot of phosphorus removal vs. time (Fig. 9), it was seen that phosphorus was rapidly removed in the initial stages of electrolysis. In particular, phosphorus removal could be achieved completely in a very short reaction time (within 2 min) by electrolyte addition.
4. Conclusion
The effect of electrolyte supplement on the removal of organics and nutrients in electrochemical wastewater treatment using copper electrode was evaluated. The COD removal improved remarkably upon the addition of the chloride ions, and most of the electrolysis reaction was completed within 20 min. The increase of organic oxidation rate may be explained by the participation of electro-generated hypochlorite ions during the electrochemical procedure. In the absence of added chloride ions, relatively poor oxidation of organics matters was observed. The reaction rate also increased gradually, as the Cl−/COD ratio increased. However, the highest removed mass of organic matter per mass of added electrolyte was observed at a Cl−/COD ratio of 1.
As the electrolysis progressed, the mass of by-products also increased gradually. However, a decrease in the settled volume of sludge and sludge volume index were observed after the addition of the electrolyte, implying that the settling performance of by-products improved. Therefore, the removal of by-products would be easily achieved.
The removal of ammoniacal nitrogen increased significantly after the addition of electrolyte, and was attributed to the generation of oxidizers such as hypochlorite in a shorter reaction time through the additional supplement of chloride ion. Complete phosphorus removal could be also achieved in a very short reaction time (within 2 min) after electrolyte addition, by electrocoagulation through the formation of compounds such as copper phosphate (Cu3(PO4)2).
Acknowledgements
This research was supported by Materials and Membranes (M&Ms) Co., Ltd. in Korea.