Sol-gel TiO2/Carbon Paste Electrode Nanocomposites for Electrochemical-assisted Sensing of Fipronil Pesticide
Article information
Abstract
The unique study of TiO2 sol-gel modified carbon paste electrode (CPE) nanocomposites have been developed for electrochemical sensor detecting fipronil pesticide compound. We develop the easy synthesized TiO2 via a sol-gel method and modified in CPE which applied electrochemical system as cyclic voltammetry (CV) because the concentration is proportional with current peaks. We discover the TiO2 optimal mass used of 0.1 g which is compared with 0.7 g carbon and 0.3 mL paraffin. It has high-current anodic (Ipa) of 1.13×103 μA and high-current cathodic (Ipc) −0.96×103 μA in scan rate of 0.5 V/s. The limit of detection (LOD) of fipronil has been determined of 34.0×10−5 μM in percent recovery of 0.8%. Its high-stability for lifetime TiO2-CPE nanocomposites was expressed for 13 days which mean that can be used for detecting fipronil pesticide.
1. Introduction
Organic pesticide widely used in agriculture due to high-efficiency for the eradicate plant pests. various kinds of attention in utilization pesticide because of the contaminant effect against living things [1,2]. One of pesticide used in agriculture is fipronil which is categorized as persistent organic pollutants (POPs) waste [3]. POPs is phenol pollutant waste in low-concentration where accumulated in the aquatic environment and contacted with living things. In the future, the pesticide effect will be affecting metabolism human. Especially, fipronil can be contained in liver and kidney of the animal which consumed by a human. Fipronil (5-amino-1-[2,6-dichloro-4-(trifluoro-methyl)phenyl]-4-[(trifluoromethyl)sulfinyl]-1H-pyra-zole-3-carbonitrile) that has upper limit threshold of 10 μM and lower of 0.1 μM [4]. Generally, it is used to control leaf pests such as Thrips sp. in chili plants, termite pest in palm oil, and Nilaparvata lugens pest attacking rice plant [5–7].
Need attention to detect fipronil pesticide using a new easy method for preparation, high-sensitivity, accuracy, and precise in low concentration. Several methods have been carried out to determine fipronil pesticide using biosensors [8], raman microscopy [9], Gas chromatography (GC) [10,11], and high-performance liquid chromatography (HPLC) [12,13], but these methods not effective for detecting fipronil pesticide in low concentration. In addition, the difficulty preparation sample caused a long time needed and many reagents preparation. So that, we attend to fabricate sensor device based titanium dioxide (TiO2) because of its high-advantages in semiconductor and photocatalyst properties [14]. Especially in this study, we made TiO2 nanoparticles using organometal solution applied in a sol-gel method due to has an advantages i.e a uniform structure, easy for preparation, and immobilization [15–17]. Various applications TiO2 sol-gel have been developed due to it can be used to fabricate electrochemical sensors based on intrinsic properties, large surface area, good conductivity, and high adsorptive properties [18,19]. TiO2 nanoparticles can be incorporated into carbon paste matrices which contribute to increasing electron transfer kinetics, providing many active sites and increasing electrode stability [20–22]. In addition, we choose carbon because it has a role of high transfer electron, the active surface morphology, and the environment-friendly. In other hands, TiO2 to high potential electron produced and response sensing electrochemical system [14,23,24].
In recent years, researchers have developed TiO2 nanoparticles for photo-sensing [25], photoelectro-chemical [26], a new material for the environment [27], self-cleaning, and solar cell [28]. These applications based on the high unique properties TiO2 like inert and high-stability in physicochemical, but in application TiO2 needed the material doping for delivering the high-stability electron transfer in the system [29–32]. One of the high-conductor properties is carbon (graphite) due to malleable, inert and light characteristics [33,34].
The TiO2 nanoparticle modified CPE has been developed as sensing hazardous organic compound and ion metals in the aquatic environment. Several studies the application of TiO2 modified carbon such as Ensafi et al. [14] has fabricated ion liquid-TiO2 nanoparticle modified CPE for determining benserazide, Hou et al. [35] TiO2 decorated carbon paper for vanadium redox battery, and Yin et al. [36] reported fipronil determination can be applied as electrochemiluminescence against potential voltage. Herein, we perform the combination TiO2-CPE nanocomposites to fabricate the electrode and its sensing of fipronil pesticide in the aquatic environment.
2. Experimental
2.1 Preparation working electrode
The organometal solution to produce TiO2 is titanium tetra-isopropoxide (TTIP) which is mixed by ethanol 99%, acetylacetonate, and acetate acid. These material refluxed at a temperature of 80°C for 3 hours. Subsequently, sol TiO2 was calcined at 500°C for 3 hours to form TiO2 anatase crystal. This crystal is crushed by agate mortal and sieved to obtain smooth the TiO2 nanoparticles [37]. Then, TiO2 was variated to obtain the mass optimation i.e. 0.01 g, 0.05 g, and 0.1 g. Then, it was mixed by 0.7 g carbon and 0.3 mL paraffin oil (d = 0.88 g.cm−3) followed heating at the temperature of 80°C to sticky between particles. Mobilization in wall glass, we rinse the probe glass by distilled water and acetone followed sonification for 15 minutes. Finally, the material was entered in probe glass with diameter 3 mm, connected by Cu wire as a conductor and also tip electrode was polished [2].
2.2 Determination of fipronil
Voltammetric detection of fipronil was conducted using a potentiostat (DY2100B-Digi.Ivy) with three electrodes system where the TiO2-CPE nanocomposites as the working electrode. Meanwhile, a platinum (Pt) and Ag/AgCl wires were used as the counter and reference electrodes, respectively. The optimation response electrode determined by using K3[Fe(CN)6] that role for increasing a solution's redox potential (E°' ~ 436 mV at pH 7). In addition, the sample tests under 1.0 M (NH4)2SO4 electrolyte due to is a strong electrolyte, stability in osmotic effect, and dissolves 100% in aqueous solution. The fipronil concentration was applied in 10−6, 10−5,10−4,10−3,10−2, and 10−1 μM to determine the linearity areas and LOD. The real sample was tested by mixing electrolyte solution for identifing peak current characteristic fipronil in the real sample. We also determination of repeatibility for 30 times and tested lifetime electrode for 23 days.
2.3 Characterization materials
The morphology of the nanocomposites was examined using a Scanning Electron Microscopy JEOLJSM-6510LV. Meanwhile, the phase and crystallinity properties were characterized via X-ray diffraction spectroscopy (XRD) using Philips X-ray diffraction spectrometer with a CuKα irradiation (λ=0.154 nm) and scan rate as low as 2° min−1.
3. Results and Discussion
3.1 Characterization of anatase TiO2-CPE nanocomposites
The anatase TiO2-CPE nanocomposites has been successfully prepared via sol-gel method. Due to phase purity of anatase TiO2 has a high-stability and electron transfer in the electrochemical system. Fig. 1 exhibits the XRD pattern anatase TiO2, there are at least 9 peaks spectrum characteristics based on JCPDS file no. 21-1272, namely 25°, 38°, 48°, 52°, 54°, 64°, 69°, 70°, and 76° with Bragg diffraction at the crystal field of (101), (004), (200), (105) (211), (204), and (215), respectively. These data have been compared with TiO2 P25 standard [2].
We then observed the anatase TiO2-CPE nanocomposites using SEM instrument. The unique microstructures were displayed that the anatase TiO2 successfully incorporated in carbon matrix (Fig. 2). In same times, high-contacted a solid-state reaction by adding paraffin as attach agent. We can conclude the anatase TiO2 nanocomposites were homogeneously distributed in the carbon paste matrix. The TiO2 given shine characteristics caused the inducing of electron to make a high-atom number easy for emitting energy. We discover the approximate size of TiO2 nanoparticles in this study is 2 μm (Fig. 2).
3.2 Mass optimization of anatase TiO2-CPE
The mass optimation aims to synergistic the anatase TiO2 combined with carbon paste which applied electrochemical system. The high current response observed based on CV by variating mass of 0.01 g, 0.05 g, and 0.1 g TiO2 sol-gel mixed pristine carbon in 0.1M K3[Fe(CN)6] as an electrolyte solution under scan rate of 0.1 V/s. The K3[Fe(CN)6] as naturally for increased separation of cathodic and anodic peaks and reversible characteristic for redox reaction. As Fig. 3, we discover the mass optimum TiO2 anatase of 0.1 g with oxidation peak the Ipa value of 1.13×103 μA, Epa value of 0.1 V with reduction peak of Ipc value of −0.96×103 μA and Epc value of 0.34 V.
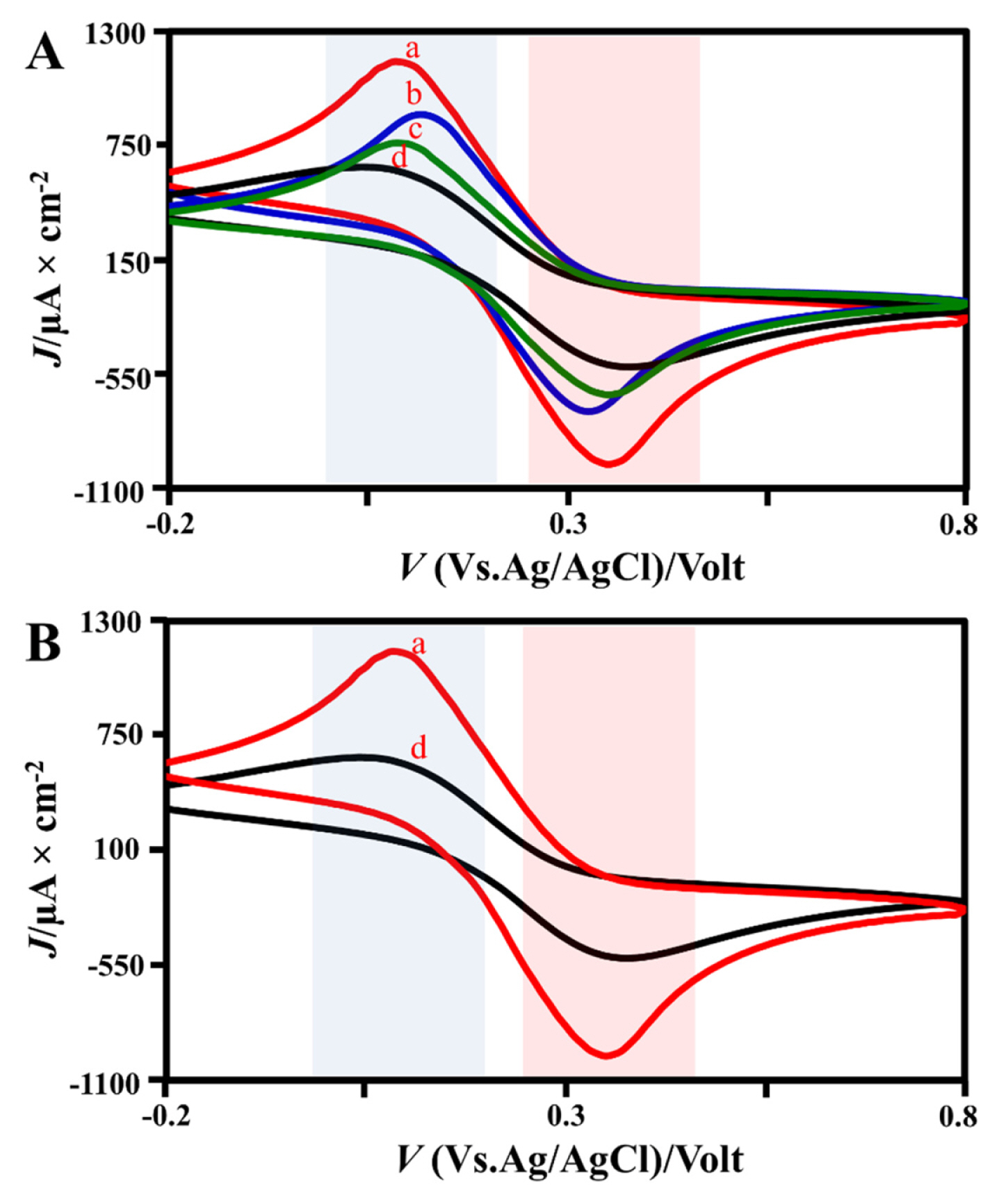
Electrochemical profile on TiO2 mass variation, (A) The electrochemical TiO2-CPE combined by the all mass variation, and (B) comparison of CPE with 0.1 g TiO2-CPE [(a) 0.1 g, (b) 0.05 g, (c) 0.01 g, (d) CPE 0.7 g]
The high composition of anatase TiO2 into the CPE matrix to increase performance the high TiO2 concentration estabilized the optimum condition. Based on Fig. 3, the sample demonstrates an excellent electrochemical response with a high current peak. In addition, it can be remarked that the electron transfer process is extremely fast as indicated by a remarkably small difference in the redox potential. The electrode with a composition of 0.1 gram TiO2 was the best electrode performance in electrochemical system causes the produce a maximum peak flow response when compared to other compositions. Finally, it is suitable to be used for measuring fipronil pesticide.
3.3 Electrochemical Response against Fipronil
This parameter was conducted to compare electrochemical response of fipronil which is containing electrolyte and without the fipronil solution. The electrochemical profile can be seen in Fig. 4. Based on the measurement (see Fig. 4) exhibit that the determination of the electrochemical response without the fipronil did not reveal peak response if it compared with adding fipronil causes the (NH4)2SO4 electrolyte solution did not react with analytes, stable, and high-electron transfer [38]. We discover the high-current response for 0.1 μM fipronil containing electrolyte has a good oxidation response with Ipa value of 31.4 μA and Epa value of −0.15 V. The Ipa value was produced based on electrode measurement responded fipronil pesticide which is an oxidation reaction.
3.4 Optimation Scan Rate Test
The optimation the scan rate has been conducted by variating the of 0.05; 0.1; 0.2; and 0.5 V/s due to the faster scan rate in analyte measurements will be produced the high-current peak. The 1.0 μM fipronil shows the measurement rate has been increased when the high variation scan rate. This is due to the scan rate effect was reversibility concentration indirectly by measuring the apparent a standard rate constant for electron transfer from only cathodic (or anodic) polarization. In a few cases both cathodic and anodic [39]. Based on analyzed (Fig. 5), we obtain the optimum scan rate on 0.5 V/s with the Ipa value of 31.7 μA and the Epa value of −0.15 V. The high scan rate has been produced proportional with the high scan rate.
3.5 Determination of Linearity and Limit of Detection (LOD)
The linearity of fipronil has been determined by plotting the variation concentration (10−6, 10−5, 10−4, 10−3, 10−2, and 10−1 μM) which is containing 1.0 M (NH4)2SO4 as electrolyte between Ipa value which produced from CV profile. Fig. 6 exhibits that the high concentration causes a high CV response. This phenomenon because of increasing fipronil concentration makes the increase ions produced to interact on the electrode surface. Based on the curve, the cathodic (reduction) potential not significantly produced because the (NH4)2SO4 electrolyte containing fipronil only giving the oxidation response onto the working electrode (see Fig. 4). The TiO2-CPE nanocomposites play a role for oxidation process sensing and high transfer electron in the electrochemical system. We then plot the linear line equation between Ipa value with concentration to obtain the linearity area from the working electrode by the range of electrode performance detecting fipronil compound in low concentrations from 10−6, 10−5, and 10−4 μM. The unique performance is identified as the LOD in low concentration which is cannot be analyzed by highinstrumentation. The higher concentration proportional with increase in anodic current [40,41]. High concentration causes more fipronil ions to accumulate on the electrode surface so that the current result is also higher. We discover the determination of LOD value was 34.0×10−5 μM by multiplying 3 with standard deviation divided by the value of the slope. This phenomenon to obtain the lowest quantity of fipronil concentration that can be identified from the working electrode.
4. Real Sample Test
We testing the TiO2-CPE nanocomposites for sensing against the real sample which is commercial pesticide in society. This due to we attempt comparing the real sample with the sample treatment 1.0 μM fipronil which aims to how are this electrode can be determining fipronil compound at the real sample. Based on this experiment, the oxidation peak has been obtained from the real sample with Ipa value of 120 μA and an Epa value of −0.1 V. Meanwhile, the 1.0 μM fipronil also shows the low oxidation peak of fipronil. We can determine the real sample concentration by comparing the Ipa value of the real sample with the 0.1 M fipronil (Ipa value = 49.1 μA) so that we obtain the real sample concentration of 0.25 μM. Based on this experiment, we concluded that the TiO2-CPE nanocomposites have a high-performance for sensing fipronil pesticide compound under low concentration.
4.1 Electrode Repeat Test
The repeatability test was conducted to exhibit the working electrode performance against the consistency of measurement. This treatment the TiO2-CPE nanocomposites has been tested by using 1.0 μM fipronil containing 1.0 M (NH4)2SO4 as the electrolyte. Fig. 8 depicts the results of repeatability working electrode that the high-performance of TiO2-CPE stability for 30 times tested. The rate electrons transfer were stabilized on the electrode surface and oxidizing the fipronil compound. Based on this study (Fig. 8), we obtain the Ipa value of 27.4 μA and calculating the standard of deviation (SD) of 2.512 and Relative Standard Deviation (RSD) is 0.8%. We declare that the TiO2-CPE nanocomposites has stabilized due to the Horwitz value is obtained less than 2%.
4.2 Electrode lifetime Determination
Especially, we explore a lifetime of TiO2-CPE to obtain the high-performance electrode for 23 days. The lifetime test performance has been carried out by using the same solution on the repeatability test. Extension lifetime electrode enforced for clarifying TiO2-CPE to sensitivity against fipronil. Fig. 9 exhibits that the optimum performance electrode from 1 until 13 days relatively stable, while the 14 until 23 days the low-performance electrode. This condition that the longer electrode used causes thickening diffusion on the electron transfer system and limiting peak current. In addition, it has saturated on electrode pores causes the current diffusion. Meanwhile, if it will be enhanced the TiO2-CPE nanocomposites should be annealing to remove the organic compound on the electrode surface.
5. Conclusions
The performance of anatase TiO2-CPE nanocomposites in voltammetric technique for examining the fipronil pesticide exhibits that the best composition of anatase TiO2 as a modifier on CPE with a composition of 0.1 gram. In addition, the voltammogram data shows an Ipa value of 1.13×103 μA, Epa value of 0.1 V with reduct ion peak of Ipc value of −0.96×103 μA and Epc value of 0.34 V. The LOD has been determined of 34.0×10−5 μM which is much lower than the fipronil maximum residual limit (MRL) in the environment, that has the upper threshold limit of 10 μM and lower of 0.1 μM. The electrode system also shows a percent recovery (% RSD) of 0.8% so that the anatase TiO2-CPE precision level is quite good and high-stability for lifetime TiO2-CPE electrode was expressed for 13 days which mean that can be used for detecting fipronil pesticide. In testing the real sample, anatase TiO2-CPE nanocomposites showed the ability to detect fipronil levels of 0.25 μM, so that this electrode is feasible to be applied to the environment.
Acknowledgement
The authors acknowledge the financial support from DRPM-Ministry of Research, Technology and Higher Education, the Republic of Indonesia under Grant No. 056/SP2H/LT/DRPM/2018.